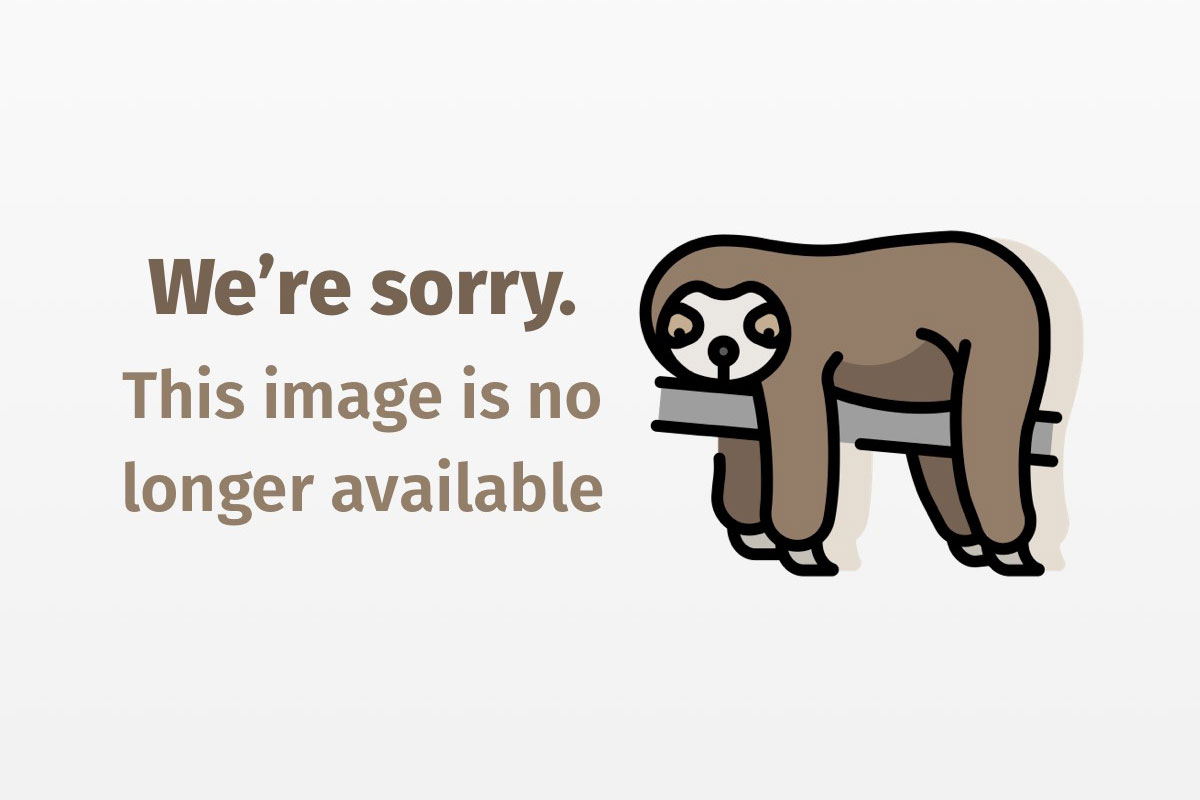
Build your own languages with JavaCC
JavaCC makes it a snap to write your own compiler or interpreter for languages of your own design in Java
Do you ever wonder how the Java compiler works? Do you need to write parsers for markup documents that do not subscribe to standard formats such as HTML or XML? Or do you want to implement your own little programming language just for the heck of it? JavaCC
allows you to do all of that in Java. So whether you’re just interested in learning more about how compilers and interpreters work, or you have concrete ambitions of creating the successor to the Java programming language, please join me on this month’s quest to explore JavaCC
, highlighted by the construction of a handy little command-line calculator.
Compiler construction fundamentals
Programming languages are often divided, somewhat artificially, into compiled and interpreted languages, although the boundaries have become blurred. As such, don’t worry about it. The concepts discussed here apply equally well to compiled as well as interpreted languages. We will use the word compiler below, but for the scope of this article, that shall include the meaning of interpreter.
Compilers have to perform three major tasks when presented with a program text (source code):
- Lexical analysis
- Syntactic analysis
- Code generation or execution
The bulk of the compiler’s work centers around steps 1 and 2, which involve understanding the program source code and ensuring its syntactical correctness. We call that process parsing, which is the parser’s responsibility.
Lexical analysis (lexing)
Lexical analysis takes a cursory look at the program source code and divides it into proper tokens. A token is a significant piece of a program’s source code. Token examples include keywords, punctuation, literals such as numbers, and strings. Nontokens include white space, which is often ignored but used to separate tokens, and comments.
Syntactic analysis (parsing)
During syntactic analysis, a parser extracts meaning from the program source code by ensuring the program’s syntactical correctness and by building an internal representation of the program.
Computer language theory speaks of programs, grammar, and languages. In that sense, a program is a sequence of tokens. A literal is a basic computer language element that cannot be further reduced. A grammar defines rules for building syntactically correct programs. Only programs that play by the rules defined in the grammar are correct. The language is simply the set of all programs that satisfy all your grammar rules.
During syntactic analysis, a compiler examines the program source code with respect to the rules defined in the language’s grammar. If any grammar rule is violated, the compiler displays an error message. Along the way, while examining the program, the compiler creates an easily processed internal representation of the computer program.
A computer language’s grammar rules can be specified unambiguously and in their entirety with the EBNF (Extended Backus-Naur-Form) notation (for more on EBNF, see Resources). EBNF defines grammars in terms of production rules. A production rule states that a grammar element — either literals or composed elements — can be composed of other grammar elements. Literals, which are irreducible, are keywords or fragments of static program text, such as punctuation symbols. Composed elements are derived by applying production rules. Production rules have the following general format:
GRAMMAR_ELEMENT := list of grammar elements
| alternate list of grammar elements
As an example, let’s look at the grammar rules for a small language that describes basic arithmetic expressions:
expr := number
| expr '+' expr
| expr '-' expr
| expr '*' expr
| expr '/' expr
| '(' expr ')'
| - expr
number := digit+ ('.' digit+)?
digit := '0' | '1' | '2' | '3' | '4' | '5' | '6' | '7' | '8' | '9'
Three production rules define the grammar elements:
expr
number
digit
The language defined by that grammar allows us to specify arithmetic expressions. An expr
is either a number or one of the four infix operators applied to two expr
s, an expr
in parenthesis, or a negative expr
. A number
is a floating-point number with optional decimal fraction. We define a digit
to be one of the familiar decimal digits.
Code generation or execution
Once the parser successfully parses the program without error, it exists in an internal representation that is easy to process by the compiler. It is now relatively easy to generate machine code (or Java bytecode for that matter) from the internal representation or to execute the internal representation directly. If we do the former, we are compiling; in the latter case, we talk about interpreting.
JavaCC
JavaCC
, available for free, is a parser generator. It provides a Java language extension for specifying a programming language’s grammar. JavaCC
was developed initially by Sun Microsystems, but it’s now maintained by MetaMata. Like any decent programming tool, JavaCC
was actually used to specify the grammar of the JavaCC
input format.
Moreover, JavaCC
allows us to define grammars in a fashion similar to EBNF, making it easy to translate EBNF grammars into the JavaCC
format. Further, JavaCC
is the most popular parser generator for Java, with a host of predefined JavaCC
grammars available to use as a starting point.
Developing a simple calculator
We now revisit our little arithmetic language to build a simple command-line calculator in Java using JavaCC
. First, we have to translate the EBNF grammar into JavaCC
format and save it in the file Arithmetic.jj
:
options
{
LOOKAHEAD=2;
}
PARSER_BEGIN(Arithmetic)
public class Arithmetic
{
}
PARSER_END(Arithmetic)
SKIP :
{
" "
| "r"
| "t"
}
TOKEN:
{
< NUMBER: (<DIGIT>)+ ( "." (<DIGIT>)+ )? >
| < DIGIT: ["0"-"9"] >
}
double expr():
{
}
{
term() ( "+" expr() | "-" expr() )*
}
double term():
{
}
{
unary() ( "*" term() | "/" term() )*
}
double unary():
{
}
{
"-" element() | element()
}
double element():
{
}
{
<NUMBER> | "(" expr() ")"
}
The code above should give you an idea on how to specify a grammar for JavaCC
. The options
section at the top specifies a set of options for that grammar. We specify a lookahead of 2. Additional options control JavaCC
‘s debugging features and more. Those options can alternatively be specified on the JavaCC
command line.
The PARSER_BEGIN
clause specifies that the parser class definition follows. JavaCC
generates a single Java class for each parser. We call the parser class Arithmetic
. For now, we require only an empty class definition; JavaCC
will add parsing-related declarations to it later. We end the class definition with the PARSER_END
clause.
The SKIP
section identifies the characters we want to skip. In our case, those are the white-space characters. Next, we define the tokens of our language in the TOKEN
section. We define numbers and digits as tokens. Note that JavaCC
differentiates between definitions for tokens and definitions for other production rules, which differs from EBNF. The SKIP
and TOKEN
sections specify this grammar’s lexical analysis.
Next, we define the production rule for expr
, the top-level grammar element. Notice how that definition markedly differs from the definition of expr
in EBNF. What’s happening? Well, it turns out that the EBNF definition above is ambiguous, as it allows multiple representations of the same program. For example, let us examine the expression 1+2*3
. We can match 1+2
into an expr
yielding expr*3
, as in Figure 1.

Or, alternatively, we could first match 2*3
into an expr
resulting in 1+expr
, as shown in Figure 2.

With JavaCC
, we have to specify the grammar rules unambiguously. As a result, we break out the definition of expr
into three production rules, defining the grammar elements expr
, term
, unary
, and element
. Now, the expression 1+2*3
is parsed as shown in Figure 3.

From the command line we can run JavaCC
to check our grammar:
javacc Arithmetic.jj
Java Compiler Compiler Version 1.1 (Parser Generator)
Copyright (c) 1996-1999 Sun Microsystems, Inc.
Copyright (c) 1997-1999 Metamata, Inc.
(type "javacc" with no arguments for help)
Reading from file Arithmetic.jj . . .
Warning: Lookahead adequacy checking not being performed since option LOOKAHEAD
is more than 1. Set option FORCE_LA_CHECK to true to force checking.
Parser generated with 0 errors and 1 warnings.
The following checks our grammar definition for problems and generates a set of Java source files:
TokenMgrError.java
ParseException.java
Token.java
ASCII_CharStream.java
Arithmetic.java
ArithmeticConstants.java
ArithmeticTokenManager.java
Together these files implement the parser in Java. You can invoke this parser by instantiating an instance of the Arithmetic
class:
public class Arithmetic implements ArithmeticConstants
{
public Arithmetic(java.io.InputStream stream) { ... }
public Arithmetic(java.io.Reader stream) { ... }
public Arithmetic(ArithmeticTokenManager tm) { ... }
static final public double expr() throws ParseException { ... }
static final public double term() throws ParseException { ... }
static final public double unary() throws ParseException { ... }
static final public double element() throws ParseException { ... }
static public void ReInit(java.io.InputStream stream) { ... }
static public void ReInit(java.io.Reader stream) { ... }
public void ReInit(ArithmeticTokenManager tm) { ... }
static final public Token getNextToken() { ... }
static final public Token getToken(int index) { ... }
static final public ParseException generateParseException() { ... }
static final public void enable_tracing() { ... }
static final public void disable_tracing() { ... }
}
If you wanted to use this parser, you must create an instance using one of the constructors. The constructors allow you to pass in either an InputStream
, a Reader
, or an ArithmeticTokenManager
as the source of the program source code. Next, you specify the main grammar element of your language, for example:
Arithmetic parser = new Arithmetic(System.in);
parser.expr();
However, nothing much happens yet because in Arithmetic.jj
we’ve only defined the grammar rules. We have not yet added the code necessary to perform the calculations. To do so, we add appropriate actions to the grammar rules. Calcualtor.jj
contains the complete calculator, including actions:
options
{
LOOKAHEAD=2;
}
PARSER_BEGIN(Calculator)
public class Calculator
{
public static void main(String args[]) throws ParseException
{
Calculator parser = new Calculator(System.in);
while (true)
{
parser.parseOneLine();
}
}
}
PARSER_END(Calculator)
SKIP :
{
" "
| "r"
| "t"
}
TOKEN:
{
< NUMBER: (<DIGIT>)+ ( "." (<DIGIT>)+ )? >
| < DIGIT: ["0"-"9"] >
| < EOL: "n" >
}
void parseOneLine():
{
double a;
}
{
a=expr() <EOL> { System.out.println(a); }
| <EOL>
| <EOF> { System.exit(-1); }
}
double expr():
{
double a;
double b;
}
{
a=term()
(
"+" b=expr() { a += b; }
| "-" b=expr() { a -= b; }
)*
{ return a; }
}
double term():
{
double a;
double b;
}
{
a=unary()
(
"*" b=term() { a *= b; }
| "/" b=term() { a /= b; }
)*
{ return a; }
}
double unary():
{
double a;
}
{
"-" a=element() { return -a; }
| a=element() { return a; }
}
double element():
{
Token t;
double a;
}
{
t=<NUMBER> { return Double.parseDouble(t.toString()); }
| "(" a=expr() ")" { return a; }
}
The main method first instantiates a parser object that reads from standard input and then calls parseOneLine()
in an endless loop. The method parseOneLine()
itself is defined by an additional grammar rule. That rule simply defines that we expect every expression on a line by itself, that it is OK to enter empty lines, and that we terminate the program if we reach the end of the file.
We have changed the return type of the original grammar elements to return double
. We perform appropriate calculations right where we parse them and pass calculation results up the call tree. We also have transformed the grammar element definitions to store their results in local variables. For instance, a=element()
parses an element
and stores the result in the variable a
. That enables us to use the results of parsed elements in the code of the actions on the right-hand side. Actions are blocks of Java code that execute when the associated grammar rule has found a match in the input stream.
Please note how little Java code we added to make the calculator fully functional. Moreover, adding additional functionality, such as built-in functions or even variables is easy.
Conclusion
JavaCC
, a parser generator for Java, makes writing parsers for programming languages a snap. JavaCC
provides a high-level notation for defining grammars, and a concise specification for grammars and associated actions. It’s also easy to read. Especially useful for Java programmers are the Java grammars that are part of the JavaCC
distribution. (The Java 1.2 grammar is a separate download.) Based on those grammars you can create your own set of nifty Java tools such as your own version of javadoc
, a Java interpreter, or a Java printer. There really is no limit to what you can do.
JavaCC
includes two additional tools. JJDoc
automatically generates documentation in HTML for your grammar in a fashion similar to javadoc
. JJTree
automatically generates actions that build a tree structure when parsing a program. It provides a framework based on the Visitor design pattern that allows you to traverse the parse tree in memory.